Concepts
- The term magnetic effect of electric current means that an electric current flowing in a wire produces a magnetic field around it.
- Hans Christian Oersted (1777-1851): Oersted showed that electricity and magnetism are related to each other. His research later used in radio, television etc. The unit of magnetic field strength is named Oersted in his honour.
- Electromagnetism: In 1820, Oersted performed an experiment to show that a current flowing through a wire produces a magnetic field around it.
- In this experiment, he found that when a magnetic needle was placed under a wire having no current flowing through it, the needle remained parallel to the wire as shown in the figure.
-
- But when electric current was allowed to flow through it by connecting it with a battery, a deflection was observed in the magnetic needle showing that a current carrying wire produces a magnetic field around it, which lasts as long as the current is flowing through the wire.
- This phenomenon is referred to as electromagnetism.
- Magnet: A magnet is an object, which attracts pieces of iron, steel, nickel and cobalt. It has two poles at ends – South and North Pole.
➢ Like magnetic poles repel each other.
➢ Unlike magnetic poles attract each other. - Magnetic Field : The space surrounding a magnet in which the force of attraction and repulsion is exerted is called a magnetic field.
- Magnetic Field Lines: A field line is the path along which a hypothetical free north pole would tend to move. The direction of the magnetic field at a point is given by the direction that a north pole placed at that point would take.
Properties of Magnetic Field Lines
- A magnetic field lines originate from north pole and end at its south pole.
- A magnetic field line is a closed and continuous curve.
- The magnetic field lines are closer near the poles of a magnet where the magnetic field is strong and farther apart where the magnetic field is weak.
- The magnetic field lines never intersect each other.
- A uniform magnetic field is represented by parallel and equidistant field lines.
- Field lines are shown closer together where the magnetic field is greater.
- Magnetic field is a vector quantity.
Magnetic Field Due To a Current through A Straight Conductor
The magnetic field lines around a straight conductor carrying current are concentric circles whose centres lies on the wire.
The magnitude of magnetic field produced by a straight current carrying wire at a point-
- directly proportional to current passing in the wire.
- inversely proportional to the distance of that point from the wire.
Right-Hand Thumb Rule
When a current-carrying straight conductor is holding in right hand such that the thumb points towards the direction of current. Then fingers will wrap around the conductor in the direction of the field lines of the magnetic field, as shown in below figure. This is known as the right-hand thumb rule. Also known as Maxwell’s Right Hand Thumb Rule.
Magnetic Field Due To a Current through a Circular Loop
- The magnetic field lines are circular near the current carrying loop. As we move away, the concentric circles becomes bigger and bigger.
- At the centre, the lines are straight.
- At the centre, all the magnetic field lines are in the same direction due to which the strength of magnetic field increase.
- The strength of the magnetic field can be increased by taking a circular coil consisting of a number of turns of insulated copper wire closely wound together.
- If a circular coil has 'n' turns, the magnetic field produced by this current-carrying circular coil will be 'n' times as large as that produced by a circular loop of a single turn of wire
The magnetic of magnetic field produced by a current carrying circular loop at its centre is
- directly proportional to the current passing.
- B ∝ I
- Where , B−Magnitude of the magnetic field , I−Current
- inversely proportional to the radius of the circular loop.
- B ∝ 1/r
- Where , B−Magnitude of the magnetic field r−Radius of the circular wire
Magnetic field due to a current in a solenoid
- A coil with number of circular turns of insulated copper wire covered closely in the shape of a cylinder is called a Solenoid.
- The solenoid is from greek word for channel.
- The solenoid is a long coil containing a large number of close turns of insulated copper wire.
- The magnetic field produced by a current carrying solenoid is similar to the magnetic field produced by a bar magnet.
- The current in each turn of a current carrying solenoid flows in the same direction due to which the magnetic field produced by each turn of the solenoid ads up, giving a strong magnetic field inside the solenoid.
Applications:
- The strong magnetic field produced inside a current-carrying solenoid can be used to magnetise a piece of magnetic material like soft iron, when placed inside the solenoid.
- The magnet thus formed is called an electromagnet.
The strength of magnetic field produced by a carrying current solenoid depends on
- number of turns(n)
- strength of current(I)
- nature of core material used in solenoid – use of soft iron as core in a solenoid produces the strongest magnetism.
Electromagnets and Permanent Magnets
- An electromagnet is a temporary strong magnet and is just a solenoid with its winding on soft iron core.
- A permanent magnet is made from steel. As steel has more retentivity than iron, it does not lose its magnetism easily.
- Increasing the current flowing through it,
- Increasing the number of turns on the coil per unit length,
- Inserting a soft iron core into the solenoid.
Why soft iron is used for making the core of an electromagnet?
- Soft iron is used for making the core of an electromagnet because soft iron loses all of its magnetism when current in the coil is switched off.
Why steel is not used for making the core of an electromagnet?
- Steel is not used for making the core of an electromagnet because steel does not loses all of its magnetism when current in the coil is switched off.
Force On a Current-Carrying Conductor in a Magnetic Field
An electromagnet is a temporary strong magnet and is just a solenoid with its winding on soft iron core. A permanent magnet is made from steel. As steel has more retentivity than iron, it does not lose its magnetism easily.
When a current carrying conductor is placed in a magnetic field it experiences a force, except when it is placed parallel to the magnetic field.
The force acting on a current carrying conductor in a magnetic field is due to interaction between:
1. Magnetic force due to current-carrying conductor and
2. External magnetic field in which the conductor is placed.
Fleming’s left-hand rule
According to Fleming's left-hand rule, stretch the thumb, forefinger and middle finger of your left hand such that they are mutually perpendicular (as shown in the figure). If the first finger points in the magnetic field direction and the second finger in the current direction, then the thumb will point in the direction of motion or the force acting on the conductor.
- Electric motors
- Electric generators
- Loudspeakers
- Microphones, and
- Measuring instruments
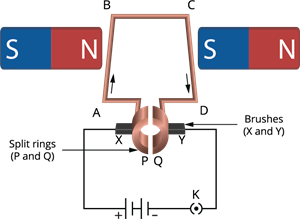
- Armature :- the armature ABCD consists of a large number of turns of insulated copper wire wound over a soft iron core.
- Field magnet :- the magnetic field (B) is supplied by a permanent magnet NS.
- Split-ring or commutator :- These are two halves of the same metallic ring(P and Q). The ends of the armature coil are connected to these halves which also rotate with the armature. The inner sides of these halves are insulated and attached to an axle.
- Brushes or sliding contacts :- these are two flexible metal plates or carbon rods X and Y which are so fixed that they constantly touch the revolving rings.
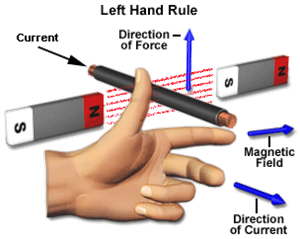
- An electromagnet replaces the permanent magnet.
- A conducting wire with a large number of turns in the current-carrying coil.
- A soft iron core on which the coil is wound.
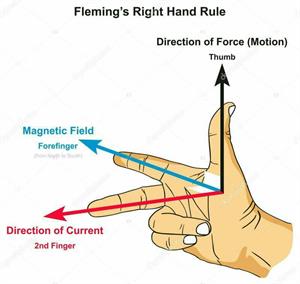
In 1831, English physicist Michael Faraday first studied this; Faraday made a significant breakthrough by discovering how a moving magnet can be used to generate electric currents.
To observe this effect, let us perform the following activity.
- Consider a coil of wire AB having a large number of turns.
- Connect the ends of the coil (AB) to a galvanometer, as shown in the below figure.
- Take a strong bar magnet and move its north pole towards the end B of the coil.
- Do you find any change in the galvanometer needle?
- Now withdraw the north pole of the magnet away from the coil.
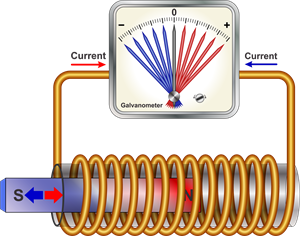
- Locate the magnet stationary at a point near the coil, maintaining its north pole towards the end B of the coil.
- When the coil is kept stationary with respect to the magnet, the deflection of the galvanometer drops to zero.
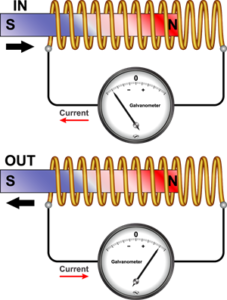
Position of a magnet
|
Deflection in galvanometer
|
Rest
|
No deflection
|
Moves towards the coil
|
Deflection in galvanometer in one direction
|
Kept stationary at the same position (near the coil)
|
No deflection in the galvanometer
|
Moves away from the coil
|
Deflection in galvanometer but in the opposite direction
|
Kept stationary at the same position (away from the coil)
|
No deflection in the galvanometer
|
- Take two different coils of copper wire with a large number of turns (say 50 and 100 turns, respectively) and insert them over a non-conducting cylindrical roll, as shown in the below figure (You may use a thick paper roll)
- Connect the coil-1, which has a larger number of turns, in series with a battery and a plug key. Also, connect the other coil-2 with a galvanometer, as shown in the figure.
- Plug in the key. Notice the galvanometer.
- Is there a deflection in the galvanometer's needle?
- Disconnect coil-1 from the battery.
Faraday's law electromagnetic induction
From that activity, we found that the motion of a magnet with respect to the coil produces an induced potential difference, which sets up an induced electric current in the circuit.
This process is called Electromagnetic induction.
Electromagnetic induction:
Electromagnetic or magnetic induction is the process of creating an Electromotive Force (EMF) or potential difference or voltage across an electrical conductor in a changing magnetic field. It is also produced when an electrical conductor is constantly moving in a stationary magnetic field.
- Number of Coils: The induced voltage or potential is directly proportional to the number of turns/coils of the wire. The greater the number of turns, the greater is the voltage or potential difference produced.
- Changing Magnetic field: Induced voltage or potential difference is affected by changing the magnetic field. This can be done by either moving the magnetic field around the fixed conductor or moving the conductor in the stationary magnetic field.
First law:
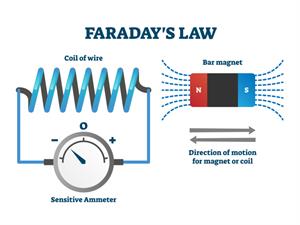
- Electromagnetic induction in AC generator
- Electrical transformers
- Magnetic flow meter
- Electric guitars and Electric violins
Galvanometer:
A galvanometer is an instrument used to detect and measure the current in an electric circuit.
Electric generators
In an electric generator, mechanical energy is used to rotate a conductor in a magnetic field to generate electricity. It works on the principle of electromagnetic induction.
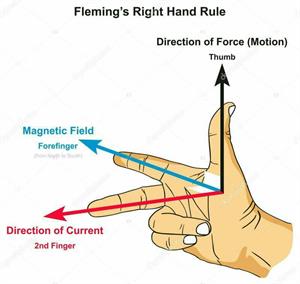
- The current rating is the maximum current that a fuse will carry for an indefinite period without the fuse element's deterioration.
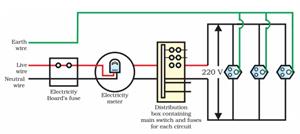
- Two different circuits should be used, one of 5 A current and the other 15 A current.
- For both 5 A and 15 A circuits, we should install the fuse.
- Parallel circuits should be used.
- Never connect too many electrical appliances at the same point.
- Never use too many electrical appliances at the same time.
- We should not connect damaged appliances in the circuit.